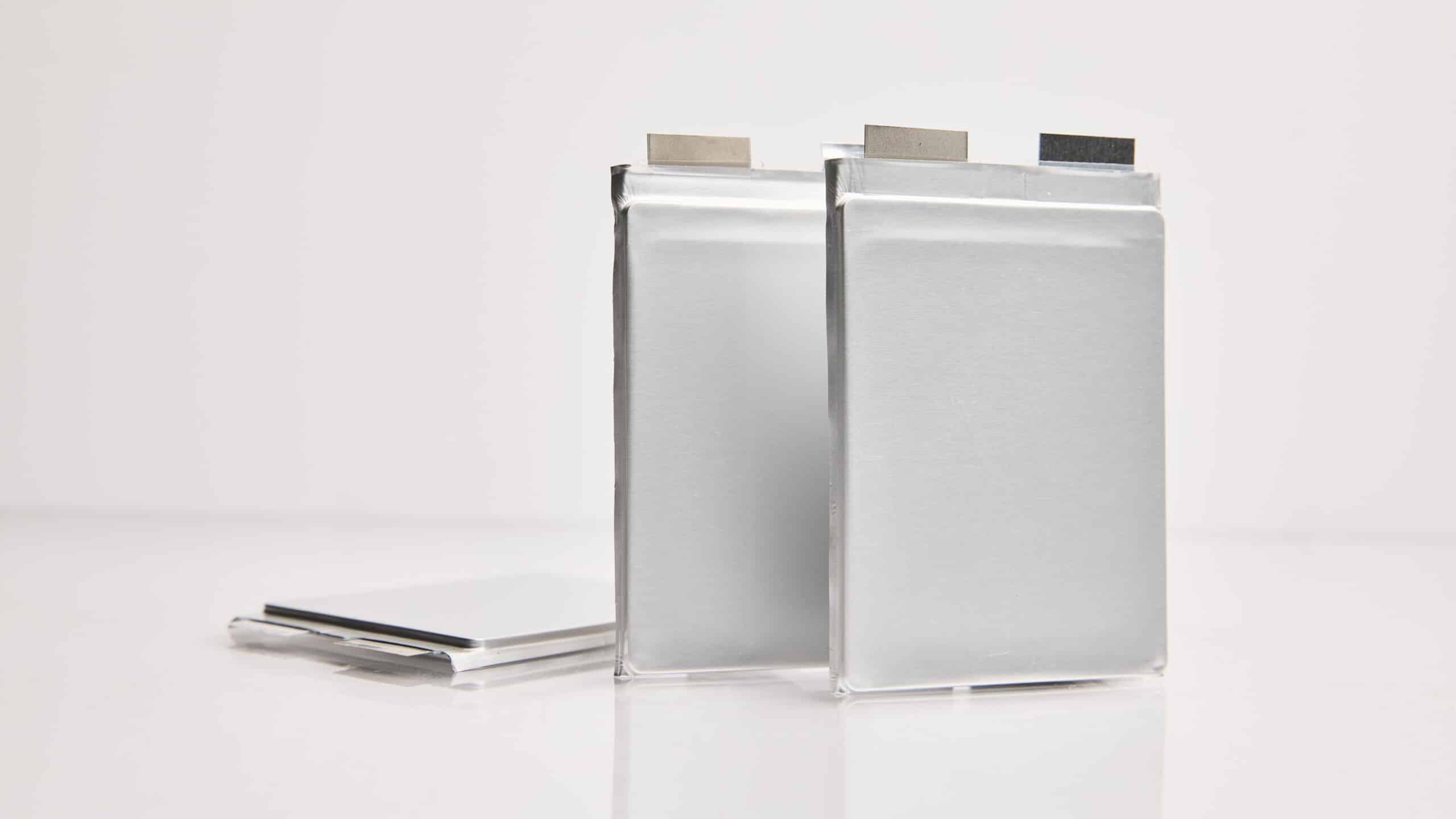
Our Strategic Blueprint
I took on the CEO role last year because I see QuantumScape as the global leader in solid state battery technologies with an ability to revolutionize energy storage and create tremendous shareholder value in the process.
As lithium-ion batteries have become ubiquitous, their safety risks have increasingly been a focus of public concern. Although electric vehicles are far less likely to catch fire than combustion-engine vehicles,[1] once a lithium-ion battery fire starts, it can be difficult to extinguish. The fire risk from conventional liquid-electrolyte lithium-ion batteries has led to more scrutiny: lithium-ion batteries cannot be stored in checked baggage on an airplane,[2] battery-powered scooters are increasingly being banned on public transportation,[3] and EV batteries have even been blamed for high-profile fires aboard cargo vessels.[4] It’s clear that, as safe as a well-made conventional lithium-ion battery may be, there is significant room for improvement.
A fire requires three elements: heat, oxygen, and some kind of fuel source. This concept is known as the fire triangle.
Imagine a Bic-style lighter. You press down on the sparkwheel with your thumb, creating a spark, a point of high localized heat, through friction between steel and flint. Your thumb lands on the button, which releases an organic hydrocarbon fuel (in this case, butane gas), which is then heated by the flint spark to ignition temperature. Lastly, the surrounding air provides oxygen that allows the initial spark to combust the butane, which in turn releases more heat energy, which will combust more butane, and so on, in self-sustaining reaction. As long as the fire is fed with more fuel and oxygen, it will continue to burn. Let go of the button and you stop the flow of fuel; douse the flame with water, and you deprive it of oxygen, smothering the reaction.
A lithium-ion battery fire is very similar to a Bic lighter, with one important difference. First, a fully charged battery has a strong electrical potential between the anode and cathode. If a battery contains a defect, such as misalignment or metal fragment, it can create a short-circuit within the battery, causing a localized hot spot equivalent to the spark from a lighter. There is also liquid electrolyte inside the battery, typically an organic hydrocarbon that is chemically quite similar to the butane in a lighter, which is potential fuel for a fire.
The key difference between a lithium-ion battery and a lighter? In a high-energy battery, the cathode active material is generally a metal oxide, such as nickel-manganese-cobalt oxide (NMC). This means that batteries contain their own oxygen and do not need to draw oxygen from the surrounding atmosphere to sustain a fire. Thus, when a conventional battery short-circuits, the result is a fire that cannot easily be extinguished, since dousing it with water does not smother the reaction. When the heat from a short-circuit causes the liquid electrolyte to react with the metal oxide cathode, the fire begins to feed on the fuel in the battery: the liquid electrolyte, the polymer separator and even the graphite anode material can all burn. As the reaction becomes fully self-sustaining, the battery enters thermal runaway.
Thermal runaway in a single battery is dangerous enough if it’s in a smartphone on an airplane, but when batteries are packed tightly together in a large pack, the problem is multiplied. Heat from a fire in one battery cell can heat its neighboring cells enough to cause them to enter thermal runaway without the need for an internal short-circuit or spark. This chain reaction from one cell throughout a battery pack is known as thermal propagation.
How can we make safer batteries?
We believe the best way to make batteries with better performance and improved safety is to replace the combustible liquid electrolyte and polymer separator that fuel the fire with a noncombustible solid electrolyte material. In the QuantumScape system, we replace the combustible polymer separator in conventional batteries with our ceramic solid-electrolyte separator. By reducing the potential fuel in the cell, fire risk can be reduced, in the same way that a lighter stops working when the butane is depleted.
However, battery safety is a highly complex problem, and many different factors can interact in unpredictable ways. A battery may be theoretically safer, but this must be demonstrated through extensive testing; no single test can prove a battery is safe. In the next part, Interpreting QuantumScape’s Safety Test Results, we examine how to evaluate battery safety and look at a few examples of different safety tests to see how QuantumScape’s technology stacks up against conventional batteries.
A common misconception about battery safety is that lithium-iron phosphate (LFP) batteries cannot catch fire. It is true that LFP offers a somewhat better safety profile than NMC-based batteries; the iron-phosphate cathode material contains oxygen, but that oxygen is trapped in a more chemically stable bond. This means that LFP can withstand somewhat higher temperatures before experiencing thermal runaway. However, LFP cells still contain the same fuels as any other conventional lithium-ion battery (liquid electrolyte, polymer separators, and graphite anode material) and still have an oxygen source in the cathode. The fact is, LFP batteries can and do experience thermal runaway, such as when exposed to high temperatures of ~200 °C.[5] And LFP batteries also require other tradeoffs, such as sacrificing energy density and hence vehicle range.
[1] https://www.kbb.com/car-news/study-electric-vehicles-involved-in-fewest-car-fires/
[2] https://www.tsa.gov/travel/security-screening/whatcanibring/all?combine=batteries
[3] https://www.electrive.com/2024/02/23/two-german-cities-place-ban-on-electric-scooters-in-public-transport/
[4] https://asia.nikkei.com/Business/Transportation/Porsche-EV-battery-blamed-for-ship-fire-in-Mitsui-O.S.K.-lawsuit
Forward-Looking Statements
This article contains forward-looking statements within the meaning of the federal securities laws and information based on management’s current expectations as of the date of this current report. All statements other than statements of historical fact contained in this article, including statements regarding the future development of QuantumScape’s battery technology, the anticipated benefits of QuantumScape’s technologies and the performance of its batteries, and plans and objectives for future operations, are forward-looking statements. When used in this current report, the words “may,” “will,” “estimate,” “pro forma,” “expect,” “plan,” “believe,” “potential,” “predict,” “target,” “should,” “would,” “could,” “continue,” “believe,” “project,” “intend,” “anticipates” the negative of such terms and other similar expressions are intended to identify forward-looking statements, although not all forward-looking statements contain such identifying words.
These forward-looking statements are based on management’s current expectations, assumptions, hopes, beliefs, intentions, and strategies regarding future events and are based on currently available information as to the outcome and timing of future events. These forward-looking statements involve significant risks and uncertainties that could cause the actual results to differ materially from the expected results. Many of these factors are outside QuantumScape’s control and are difficult to predict. QuantumScape cautions readers not to place undue reliance upon any forward-looking statements, which speak only as of the date made. Except as otherwise required by applicable law, QuantumScape disclaims any duty to update any forward-looking statements. Should underlying assumptions prove incorrect, actual results and projections could differ materially from those expressed in any forward-looking statements. Additional information concerning these and other factors that could materially affect QuantumScape’s actual results can be found in QuantumScape’s periodic filings with the SEC. QuantumScape’s SEC filings are available publicly on the SEC’s website at www.sec.gov.
I took on the CEO role last year because I see QuantumScape as the global leader in solid state battery technologies with an ability to revolutionize energy storage and create tremendous shareholder value in the process.
QuantumScape’s planned first commercial product, QSE-5, is a ~5 amp-hour cell designed to meet the requirements of automotive applications. Here, we’ll walk through the various elements of the cell specifications and explain some of the complexities behind the seemingly simple metric of energy density.
I took on the CEO role last year because I see QuantumScape as the global leader in solid state battery technologies with an ability to revolutionize energy storage and create tremendous shareholder value in the process.
QuantumScape’s planned first commercial product, QSE-5, is a ~5 amp-hour cell designed to meet the requirements of automotive applications. Here, we’ll walk through the various elements of the cell specifications and explain some of the complexities behind the seemingly simple metric of energy density.
Privacy Policy | Terms of Use
© 2025 QuantumScape Battery, Inc.
1730 Technology Drive, San Jose, CA 95110
info@quantumscape.com
Pamela Fong is QuantumScape’s Chief of Human Resources Operations, leading people strategy and operations, including talent acquisition, organizational development and employee engagement. Prior to joining the company, Ms. Fong served as the Vice President of Global Human Resources at PDF Solutions (NASDAQ: PDFS), a semiconductor SAAS company. Before that, she served in several HR leadership roles at Foxconn Interconnect Technology, Inc., a multinational electronics manufacturer, and NUMMI, an automotive manufacturing joint venture between Toyota and General Motors. Ms. Fong holds a B.S. in Business Administration from U.C. Berkeley and a M.S. in Management from Stanford Graduate School of Business.